Years from now, when we look back on today’s vaccine development scene, we might say it was almost as consequential as the transition from variolation to vaccination. Variolation, an ancient method, involved the use of infectious material from a person with smallpox to inoculate others against the disease. Dried and powdered material from pus or scabs would be blown up the nose or jabbed beneath their skin. Although variolation had a fatality risk as high as 3%, as well the potential to contribute to the spread of smallpox and other diseases, no better protection was available, not until it was overtaken by vaccination in the 19th century.
Vaccination made inoculation far safer and far more effective. Also, it came to be used against a widening array of diseases. Analogous improvements are now promised by advanced vaccine technologies. Some of these technologies—such as mRNA-based technologies—are breaking new ground. Others are updated versions of established technologies.
“Post-COVID-19, a huge portion of the technology focus has shifted to mRNA- based technologies,” says Kate Broderick, PhD, a molecular geneticist and the chief innovation officer at Maravai LifeSciences. Broderick has worked for many years on vaccines for Ebola, Zika, Lassa fever, and Middle East respiratory syndrome.
“For an infectious disease vaccine, what you really want to see is what we call neutralizing antibodies,” she continues. “The mRNA vaccines are really incredibly good at generating those particular types of antibodies. And they’re showing that they’re able to generate T-cell responses, too.”
Indeed, mRNA vaccines work so well that Broderick believes many future vaccines will likely rely on the technology. Efforts are already underway to design mRNA-based vaccines ahead of a potential flu pandemic, as well as efforts to advance the development of self-amplifying mRNA vaccines, which use small doses of synthetic RNA to generate large quantities of antigen for a robust immune response.
This technology is still in its infancy, but according to Broderick, its potential is already emerging. “Self-amplifying RNA was kind of being developed at about the same time as mRNA, but it didn’t get the focus that mRNA did during COVID-19,” she relates. “Now we’re starting to see some really, really interesting results there.”
However, mRNA vaccines are not a magic bullet. “Just because it worked so brilliantly for COVID-19 [does not mean] it’s going to work brilliantly for everything else,” Broderick cautions. “Honestly, some things have worked, and some things haven’t worked.”
That means there is plenty of room for other technologies to proliferate and thrive, including updated versions of established technologies. For example, several companies are updating live, attenuated vaccine technology by incorporating some modifications to boost safety and efficacy. Also, subunit vaccine technology, which uses purified protein antigens derived from the target pathogen, is being used to develop new vaccines for respiratory diseases by companies like Novavax.
Innovation extends to vaccine delivery technology. One such technology is the microarray or microneedle patch. It is covered with tiny needle-like projections that penetrate and deliver vaccines to the top layers of skin. Its thermostability, ease of administration, and needle-free nature makes it an attractive option, particularly in resource-constrained contexts. Also, lipid nanoparticle technology is becoming safer and more stable, helping it to become the technology of choice for delivering mRNA-based vaccines.
There are also opportunities to use artificial intelligence (AI) in vaccine design and development. For example, AI can be used to model vaccine-protein target interactions, predict immune responses, and improve manufacturing efficiency. “An area that AI is really advancing is the management of [vaccine] clinical trials,” Broderick points out. “If you are running a global infectious disease vaccine clinical trial at 10 sites in Africa and 3 sites in the United Kingdom, AI has the ability to integrate [the data] and provide feedback to the clinicians or the developers that is much more real time.”
Virus-like particle vaccines get an upgrade
Launched in 2017, Osivax has offices in both France and Belgium, two hubs of vaccine development in continental Europe. The company is using its virus-like particle (VLP) technology platform to develop a novel class of T-cell vaccines that have broad-spectrum efficacy against multiple strains of a single virus. “This technology could be applicable to quite a few respiratory viruses,” says Alexandre Le Vert, Osivax’s co-founder and CEO. The company’s first program is for a universal influenza vaccine.
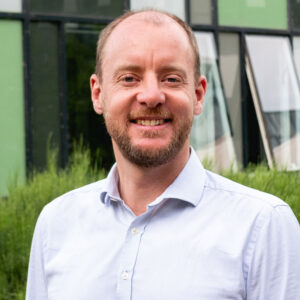
Co-Founder and CEO, Osivax
One of the biggest hurdles in vaccine development is creating vaccines that are highly efficient. Current influenza vaccines are only about 40% effective because viruses can mutate rapidly and escape immune surveillance. Osivax’s data, which has been published in Lancet Infectious Disease, shows that the company’s vaccine has a much higher efficacy than current vaccines—about 84%—against viral strains responsible for seasonal influenza.
Osivax spun off from Imaxio, another French firm. Imaxio developed OligoDOM, the VLP technology that Osivax uses to make its vaccines. Osivax describes it as a self-assembling nanoparticle platform that is designed to trigger T-cell and B-cell immune responses. VLP technology is a well-established technology, used in vaccines to protect against viruses such as human papillomavirus and hepatitis B virus. “The issue that we used to have with VLPs is that they were able to trigger a strong antibody response, but not a strong T-cell response,” Le Vert explains. “We’ve improved [the] technology to make VLPs that also trigger a very strong T-cell response.”
In the context of influenza, Osivax used OligoDOM to design and produce a recombinant version of nucleoprotein, a highly conserved internal antigen. The recombinant protein self-assembles into a nanoparticle that activates the immune system. “It’s an improved way to create nanoparticle vaccines to trigger broad protection against all the strains of a given virus,” Le Vert maintains.

To date, Osivax has tested its universal influenza vaccine in over 1,200 participants in five clinical trials in multiple countries in Europe as well as in Australia. However, the vaccine is likely still years away from commercialization. At the time of publication of this article, Osivax has an investigational new drug application for its OVX836 universal influenza vaccine with the U.S. Food and Drug Administration and has plans to launch additional trials, starting with a Phase IIb trial in 2024 followed by a third phase of testing thereafter.
In terms of the delivery mechanism, Le Vert says that Osivax’s vaccine will be given as a standard intramuscular injection to ensure that it’s easy for healthcare providers to administer and to ensure compliance from patients. Producing the vaccine on a large scale should also be straightforward. “It’s the benefit of the [VLP] technology that we’re using,” he asserts. “Manufacturing is always a very big challenge in vaccines because you have to produce millions of doses. But compared to other vaccines, we think it’s one of the strengths.”
In addition to influenza, Osivax is developing vaccines for Sarbecovirus and human papillomavirus, and is cultivating an mRNA vaccine program.
Live, attenuated vaccine revival
Clinical virologist and biopharma executive and entrepreneur Paul Grint, MD, believes that live, attenuated vaccines could play a larger role against many infections, particularly respiratory infections. “Credit to the mRNA technology and the Pfizers and Modernas of this world because they really stepped in when we had a massive global need,” he says. “Those vaccines have had a profound impact—but SARS-CoV-2 is not going away.”
Grint is entrepreneur in residence at the University of California, San Diego, and holds board-level positions in several companies, including Codagenix, which combines live, attenuated virus design with codon deoptimization to develop synthetic biology–based vaccine solutions. Using live, attenuated viruses can be advantageous against respiratory infections, facilitating intranasal delivery and promoting mucosal immune responses. Other benefits include immunity that is systemic and of long duration.
“It’s clear with some of the mRNA vaccines that you get a good antibody response but one that is relatively short lived,” Grint notes. “In the influenza setting, you’re seeing most of the infections really limited to the winter. That doesn’t seem to be the case with COVID-19. We’ve seen significant increases in late spring and early summer over the last couple of years. Promoting booster vaccines in the early fall if you’ve lost most of your immunity coverage by January or February doesn’t help if you then get potentially exposed in the late spring and early summer.”
Unlike traditional approaches to live, attenuated vaccines, the Codagenix approach leverages synthetic biology and machine learning. Whereas the traditional approaches give viruses just a few mutations, the Codagenix approach introduces hundreds of intentionally placed edits, making it probabilistically impossible for the virus to revert to the wild-type phenotype.
The other piece of the synthetic biology puzzle for Codagenix pertains to manufacturing. “Some flu vaccines are still manufactured in eggs,” Grint says. “Basically, the virus grows in the chicken embryos that are harvested, put in a blender, and then slightly purified—that’s the vaccine. The reactions to that vaccine can be very problematic, and clearly, it’s not the type of manufacturing process one would like. You can use synthetic biology to turn that into something where, based on the cell line, you can easily produce large amounts of a highly purified vaccine.”
Codagenix’s live, attenuated viruses can be effectively produced in cell culture, which does not possess adaptive immunity at commercial scale in existing manufacturing facilities, is stored in conventional cold chains, and is easily delivered to far reaches of the globe.
Another company pursuing the live, attenuated virus route, but with a different twist, is Emergex Vaccines. Founded in 2014, Emergex initially planned to develop a vaccine for the emerging Ebola epidemic in West Africa. Challenges with testing that vaccine led Emergex to shift to being prepared for, rather than reacting to, an outbreak. The company now develops vaccine constructs that can be used against some of the world’s nastiest viruses, and that can be quickly rolled out and used in future pandemics or epidemics.
The idea is to create vaccines that stop emerging viruses before they can establish themselves, explains Thomas Rademacher, MD, PhD, Emergex’s co-founder and CEO and an emeritus professor of molecular medicine at University College London. “You have to be able to make a vaccine that you have fairly good confidence is going to work,” he says, and it has to be made “cheaply enough and easily enough such that if governments want to store 50 million doses or 10 million doses … it’s not going to cost them $200 or $1,000 a dose.”
Emergex vaccines target RNA viruses, which are responsible for diseases such as Ebola, dengue, and Zika. RNA viruses exist as clouds of related sequences—sometimes called quasi-species clouds—that work cooperatively to cause infection. These clouds are highly heterogeneous with multiple viruses present, so targeting only one member may not be enough to stop the infection.
Historically, live, attenuated viruses have been the best weapon against RNA virus–based infections by recruiting armies of T cells to kill off infected cells. But these kinds of vaccines can be lethal to their host. “No regulator is going to allow you to make an attenuated Ebola vaccine and [give] it to somebody,” Rademacher states. He adds that you can get around this prohibition if you make vaccines that are not alive but can still do what live, attenuated vaccines do.
Emergex does this by extracting and sequencing viral signatures from infected cell cultures and using them to create completely synthetic attenuated vaccines that can trick the body’s immune system into responding as it would if the vaccine was alive. These synthetic vaccines prime the T cells so that they are able to mobilize and destroy infected cells within hours of the initial infection, well before the viruses have time to produce and disseminate protein throughout the body.
“Under a normal infection, it takes about six or seven days to build up a T-cell army to kill that cell,” Rademacher notes. “If I can kill that cell within the first 20 hours of infection, you have an abortive infection. It doesn’t produce any virions, and the infection doesn’t spread.”
To have the best chance at generating an army of T cells, Emergex’s vaccines are delivered using microneedle technology. “For vaccines to be effective, you have to have tissue-resident cellular immunity,” Rademacher explains. “If you vaccinate into the epidermal layer, the T cells that are generated there go on to become resident T cells all over the body, and they create systemic immunization. If you give our vaccines intramuscularly or subcutaneously, they won’t work.”
So far, Emergex has conducted multiple vaccine trials including two in Switzerland that tested a dengue vaccine and a universal coronavirus vaccine at the same time. The company is now planning a universal flu vaccine trial in the United States. Emergex is also testing vaccines in Phase II trials in the Philippines and has agreements to test four vaccines in Brazil and South America. Separately, the company has purchased a facility in Fremont, CA, where it will manufacture its own microneedles and vaccines.
This article has been updated to correct the name of company that Osivax spun off from to Imaxio rather than Axio as written previously.